Greetings, all. Please see below the 2018 Summer edition of
FGS News and Research. Our lead
article is about the oldest samples in the Florida Geological Sample Repository,
and it may not surprise you to learn the rocks came from a well in Florida’s oldest
city, St Augustine. Our “Featured
Formation” this issue is the Miami Limestone – one of the most transmissive
aquifers in the state, providing outcrops of classic sedimentary
structures. We “scan the unseen” in two
articles: first, to learn about the geophysical tools used by the FGS to explore
rocks, sediments and groundwater in Florida’s subsurface; and second, to
discover emerging contaminants - the hidden “fingerprints” that human activity
is leaving on natural waters.
Earth Science Week will be a grand event this year, and we
are excited about the theme “Earth as Inspiration.” I invite you to help us celebrate the event
by attending the FGS Open House on October 18, and join our “Earth as Inspiration”
Writing and Art Contests to win amazing prizes! Details along with more information will come
soon.
Finally, on behalf of all FGS staff, I wish a fond farewell
to Doug Calman, FGS Librarian, who retired on July 31. Doug’s history with the FGS spans decades, and
you can read more about his important geoscience contributions below.
Sincerely,
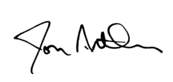 Jonathan D. Arthur, Ph.D., P.G. Director and State Geologist Florida Geological Survey Florida Department of Environmental Protection
FGS
employees Dave Paul and Guy Richardson were surprised to discover that the
oldest set of samples in the state’s Geological Sample Repository collection
are not from FGS well accession number 1 (or W-1). Instead, they found the oldest samples
recovered and archived are from W-236, which was drilled to supply water to the
Hotel Ponce de Leon and Alcazar Hotel in Florida’s oldest city, St. Augustine. The
hotels were part of the Florida East Coast Hotel Company, a subsidiary of the
better-known Florida East Coast Railway Company. Its owner - Henry Flagler.
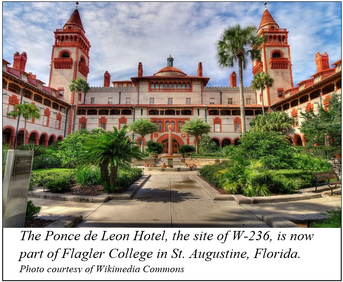
Drilling for W-236 began on November 27, 1886,
under the direction of contractor Daniel Dull from New York. The 12-inch well
was completed on February 24, 1887. The well was originally intended to be
2,000-feet deep, however, the drill bit was lost at 1,490 feet, and the driller
had encountered sulphurous and dissolved mineral-rich waters.
The
FGS
obtained samples to 1,440 feet. The artesian flow from the well produced 8 to 10
million gallons per day and sustained sufficient pressure to force the jet of
water over 20 feet above the top of the well. Interestingly, an illustration
included in an 1887 Scientific American article appears to show the fountain in
a plaza south of the Ponce de Leon Hotel. Other information suggests that W-236
was located west of the Hotel Ponce de Leon, near the north end of a swimming
pool constructed sometime after 1952.
Prior
to the 1888 opening of the Hotel Ponce de Leon, Thomas Edison’s electrician,
William Hammer, set a turbine over one of the artesian wells on the property.
The water-turbine-powered dynamo could light 88 16-candlepower lamps with a
uniform, steady glow. On April 7, 1888, as part of the extravaganzas to close
the first winter season of the hotel, he used the dynamo to light the wooden
tennis courts for an evening lawn party for 500. It is the first recorded case
of natural waterpower directly from the earth driving machinery, in this case
an electric light plant consisting of the turbine and dynamo. Subsequently, four coal-fired direct current steam dynamos, rather than power generation from the well, were employed to power the hotel for day-to-day electric lighting operations.
Due
to natural increase in temperature with depth, the well provided 82°F
water for the pool at the Alcazar Hotel (today is the Lightner Museum). The
water was also pumped into the 4,000-gallon storage tanks in the two towers of
the Hotel Ponce de Leon to provide pressure to the pistons in the basement to
operate the hydraulic elevators. Additionally, the water was used to provide
hotel plumbing for guest services. The fountain of water and four other
fountains on the property were used to help aerate the water and reduce the sulphurous
smell and remove some of the dissolved mineral content.
This
system remained in place until completion of the new water supply from Moultrie
Creek that was started in 1891 and placed into service in February 1892. The
processing and filtration of the surface waters from Moultrie Creek removed the
odor and dissolved mineral problems encountered with the artesian water
supplies. To read more, contact the author below for a complete bibliography.
Contact: Christopher P.
Williams, P.G. II
Back to top
|
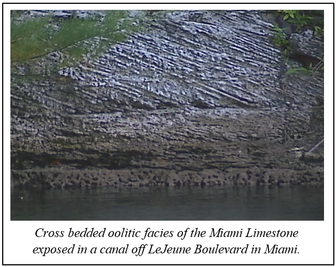
Miami oolite was the name
given by Samuel Sanford in 1909 to the white, chalky, oolitic (rounded grains) limestone
that occurs over a large portion of southeastern Florida. Sanford named and
described this lithostratigraphic unit from outcrops he observed along the
Miami River and New River and on Long Key, and designated the type locality as
being in Miami (no specific locality within Miami). Sanford described the unit as a soft, white,
oolitic limestone that contains variable amounts of quartz sand and some fossil
shells. He estimated the maximum thickness of the unit to be about 50 feet.
The oolitic limestone
that is exposed in southeastern Florida and the Keys was first reported by U.S.
Army officers during the Seminole Indian Wars in the 1830s. Prior to Sanford’s
work in 1909, several authors, including Buckingham Smith (1848), Michael Toumey
(1851) and the well-known biologist/geologist Louis Agassiz (1852), wrote
about the oolitic limestone deposits exposed in this area and speculated on its
origin and age. Some believed that the deposits were transported and deposited
by wind (called eolian processes), and others believed that the oolitic limestone
was deposited in the marine environment.
The currently recognized
name for this lithostratigraphic unit, the Miami Limestone, was proposed by J.
Hoffmeister, K. Stockman and G. Multer in their 1967 paper on the Miami
Limestone. In their paper, they recognize two facies (differences in lithology
within the same rock unit that reflect different depositional environments): an
upper oolitic facies and a lower bryozoan facies. Both facies, combined,
constitute the Miami Limestone. The Miami Limestone occurs in the southern
portion of Broward County, the eastern portion of Monroe County and across all
of Miami-Dade County. The oolitic facies of the Miami Limestone is also exposed
in the Lower Keys from Big Pine Key to Key West. The oolitic facies of the Miami Limestone is exposed along the gentle
topographic high that exists along the southeastern coast of Florida called the
Atlantic Coastal Ridge. Outcrops of the oolitic facies commonly exhibit cross
bedding reflecting the action of water currents on the ooids – the small,
round, calcium carbonate spheres that form the rock. Today, ooids are forming
in marine environments of the Bahamas. They form in shallow, warm shoal
environments where water currents constantly move hypersaline water back and
forth across the shoal.
|
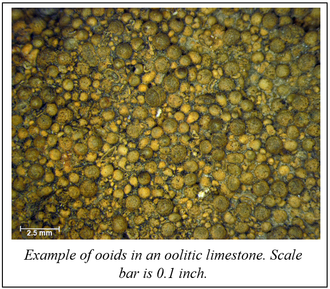
The hypersaline water carries dissolved calcium carbonate that can
precipitate when conditions are
right. The calcium carbonate
grows in concentric layers around a nucleus, which can be a sand grain or piece
of shell. The moving water keeps the newly forming ooid in the shape of a
sphere. The ooids can accumulate on shoals and, over geologic time, can become
cemented to form oolite. The modern Bahama environments where ooids are forming
today may be similar to the ones that existed in southeastern Florida when the
oolitic facies of the Miami Limestone formed. Many similarities between the
modern
Bahama
ooid shoals and the oolitic facies of the Miami Limestone have been observed
and show how modern analogs can help us understand past environments.
The bryozoan facies of
the Miami Limestone consists of large numbers of fossil remains of colonial
animals known as bryozoans. Fossil
remains of the species Schizoporella
floridana have been estimated to constitute up to 70 percent of the rock by volume
in some areas (Hoffmeister et al., 1967). Other fossils found in the bryozoan facies of the Miami Limestone
include worm tubes, mollusks and calcareous algae. The bryozoan facies is
exposed west of the Atlantic Coastal Ridge throughout much of the western
Everglades. The thickness of the bryozoan facies ranges from about 10 to 15
feet.
|
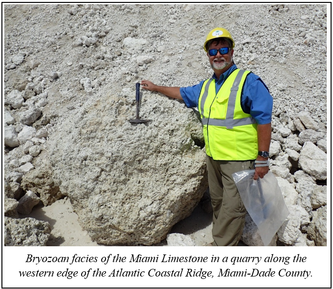
The Miami Limestone was deposited during the late Pleistocene,
approximately 130,000 years ago during the last interglacial period when sea
levels were 20 to 26 feet higher than today. The age of the Miami Limestone was determined through radiometric dating
and correlation with other strata of similar age. In southeastern Florida, the Miami Limestone
constitutes part of the Biscayne aquifer, which supplies fresh water to millions
of people.
References:
Sanford, S., 1909, The
topography and geology of southern Florida, Florida Geological Survey Second
Annual Report, 299 pages.
Hoffmeister, J.E.,
Stockman, K.W., and Multer, H.G., 1967, Miami Limestone of Florida and its
recent Bahamian counterpart, Geological Society of America Bulletin, v. 78, p.
175-190.
Contact: Harley Means, P.G. Administrator
Back to Top
|
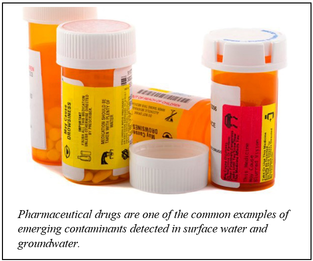 The phrase “emerging groundwater contaminants” refers to the organic compounds previously not considered or known to be significant in groundwater with regards to their concentrations, distributions and impacts. With increasing instrument sensitivity and advancement of analytical techniques, these contaminants are now being more widely detected in surface water and groundwater. Commonly known examples of emerging contaminants include pharmaceuticals, personal care products, pesticides/herbicides, fire retardants, hormones and detergents. It is important to remember that although these contaminants can be detected, they may or may not cause adverse human health and environmental impacts. Some known effects of emerging contaminants on aquatic animals include declines in reproduction, changes in male-female ratios, developmental abnormalities and reduced survival. While scientists are just beginning to understand the effects of emerging contaminants on humans, possibilities include diminished intelligence, altered behavior and development, and declining immunity to diseases.
Major sources of emerging contaminants in groundwater include domestic wastewater, pesticides and manure/sludge application to soil, treated wastewater discharge to surface water, managed aquifer recharge, septic tanks, animal waste, and landfill activities. There are several pathways for these contaminants to enter the aquatic environment. How fast and how much these contaminants reach the groundwater depends on biological, chemical and physical aspects of the surface/subsurface materials, and interconnection between surface water and groundwater. Microorganisms and organic matter in soils and sediments drive a series of biogeochemical reactions that break down and remove some of the contaminants before they reach to the groundwater. Likewise, low-permeability soils and thick, clay-rich sediments overlying a water-bearing rock or sediment layers (aquifers) help to protect groundwater from contaminants introduced at the land surface. On the other hand, much of Florida’s groundwater is highly vulnerable to contamination. Florida’s nearly ubiquitous sinkholes can capture contaminants introduced on land surface via runoff and drain these contaminants directly into groundwater. In addition, absence of protective clay-rich layers above highly porous water-bearing rocks (e.g., limestone) increases the probability of groundwater contamination with minimal filtration or benefit of microbiological processes.
The Florida Department of Environmental Protection monitors and documents potential effects of emerging contaminants in Florida’s groundwater. Recently, Seal and others (2016) noted the behavior of sucralose as an indicator of human waste pathways into the environment. Sucralose is commonly used as an artificial sweetener in a wide variety of food products. This organic compound is resistant to many wastewater treatment methods. The study detected sucralose in up to 25 percent of 116 groundwater samples from an aquifer where protective layer is absent. In addition, pharmaceutical compounds were detected in 12 percent of those wells. Given the fact that more than 90 percent of Florida’s drinking water comes from groundwater, the study highlights the importance of keeping emerging contaminants on our radar to protect Florida’s vulnerable water resources. In fact, as Florida considers water reuse, the concern of emerging contaminants is an important topic being considered by the Potable Reuse Commission.
Resource:
Seal, T., Woeber, N.A., & Silvanima, J. (2016). Using tracers to infer potential extent of emerging contaminants in Florida's groundwater. Florida Scientist, 279-289.
Contact: Dr. Mitra Khadka, Environmental Specialist III
Back to top
|
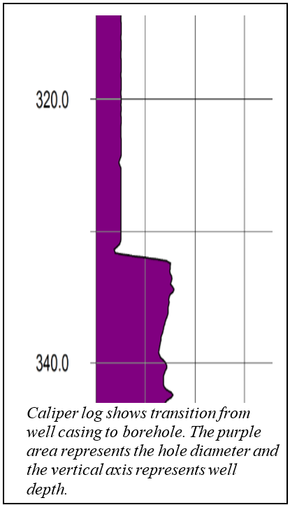
Drilling cores and
installing wells provides opportunities to learn about the rocks and groundwater
in the subsurface. Geophysical logging of these wells, or any borehole into the
earth, offers another dimension of information. By lowering specialized tools
or probes into boreholes, data about the chemical and physical properties of
rocks, sediments and water can be collected. The FGS uses several of these probes to provide its geoscientists with
important information as they interpret subsurface geology and aquifer
properties. In this article, we describe
a few of the geophysical probes in the FGS toolbox and the information they
provide.
A gamma-ray probe
measures natural background radiation emitted from rocks and sediments. The data are collected in units of counts per
second and are plotted on a graph with depth (typical format of a geophysical
log) to identify different patterns within and between geologic units. Like many probes that measure physical
properties of geologic strata, the gamma-ray probe is similar to a “fingerprint”
of the local subsurface geology. These
patterns can be compared across wells to determine the lateral extent of geologic
units that contain varying amounts of organic material, clays or phosphate. For example, sediments of the Hawthorn Group are
readily identified using a gamma-ray log. The probe is versatile because it can
collect data from an open borehole or a constructed well that already includes
a PVC or steel well casing.
The caliper probe
provides a measurement of the borehole diameter. The probe has three spring-loaded
arms that press against the borehole wall, and as the tool is retrieved up the
borehole, a record of the diameter is obtained. Caliper logs are useful in interpreting the relative resistance of the
rocks and sediments to weathering or abrasion by the drilling process. The
curve shows where the borehole dimension closely matches the drill bit’s outer
diameter, and where softer material has allowed the drilling process to wash
out the borehole to a larger diameter. It also shows where cavities or caverns
exist. Caliper logs allow a well driller to estimate the borehole volume, which
is useful in determining how much grout is needed if the well is to be
sealed and abandoned. Caliper logs are also collected after well construction
to confirm the depth and diameter of well casing and check to ensure the casing
has not been damaged during installation. This log is useful in the analysis of other logs that are influenced by
borehole diameter.
The electrical
resistivity probe involves use of an electrical current that is passed between electrodes.
The system records the relative resistance of the surrounding geologic
formation to that charge. Resistivity logs can help geoscientists interpret the
type of sediment or rock as well as the nature of fluids present and the porosity
of the formations penetrated by the borehole. Units measured by the probe are
in ohm-meters. As an example, the
presence of seawater or clay minerals would be represented by low resistivity
because seawater and most clays are electrically more conductive. The probe can collect data only in the open
hole portion of the borehole; it cannot collect data through PVC or steel well
casings.
|
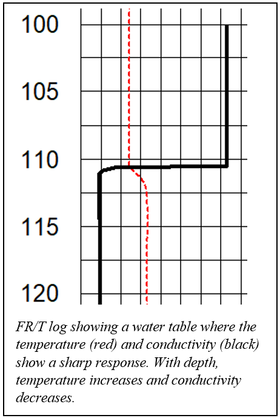
Unlike the electrical
resistivity probe, the fluid resistivity/temperature probe (FR/T) probe records
data only from the fluid in the borehole and not the surrounding formation. It
records temperature in the dry portion of the borehole and below the water
table. In fact, this tool provides an excellent measurement of the depth to the
water table because the water is always of a different temperature than the air
in the borehole, and the response on the log is very clear. This tool also
provides a measure of conductivity, which is calculated from the resistivity
data. Conductivity is a measure of how conductive the water is due to dissolved
solids such as chloride (salt) in the water. Scientists can use these data to
model different water-bearing formations, and well contractors can use the data
to decide how to construct a well that will provide safe drinking water.
Because this probe measures properties of the water in the borehole, it needs
to be run after the well has been undisturbed for a while.
|
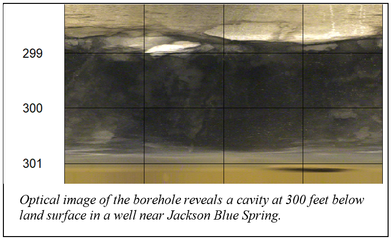 When it comes to appreciating photographs, geoscientists are no exception. The optical televiewer and borehole imager records an image of the borehole as the probe is slowly moved up or down the hole. The image is displayed as a long, flat picture of the borehole wall. In other words, imagine a 360-degree image rolled flat. This probe is a highly specialized camera, and best results are obtained after all drilling mud is cleaned out of the hole through a process called well development.
In the image above, it is likely that the groundwater in the cavity is moving. The flowmeter probe measures fluid movement in the borehole. When a hole is drilled through different water-bearing formations, these previously isolated (or confined) units are then connected and some may flow at a higher rate. The flow meter records these different flow rates and can provide scientists valuable hydrogeologic data for modeling groundwater resources. The movement of water between confined formations is generally not desirable, so after coring or well drilling is complete, the hole is abandoned by filling it from bottom to top with grout so that the confining units are restored and the layers of the aquifer are left as unaltered as possible.
The FGS has only a small number of probes, but many more exist, including water quality probes, borehole imaging probes that use sound (acoustics), and nuclear source probes. Water quality probes can be built to order for testing for specific groundwater quality parameters. Acoustic imagers record a finely detailed image of the borehole, much like the optical imager. The advantage of the acoustic imager is that it can collect data in a mudded borehole, whereas the optical probe requires clear water. Nuclear based tools can provide information on rock and sediment chemistry or porosity. These are just a few of the many geophysical probes that unlock information about the subsurface.
Contact: David Paul, P.G. Administrator
Back to top
|
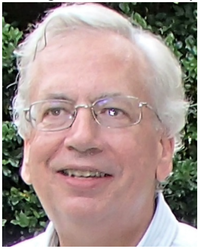
The beginning of the
fiscal year is a bittersweet time for the Florida Geological Survey as
staff bid a fond farewell to John Douglas Calman (Doug), a Librarian at FGS. After
15 years with the organization and 32 years with the state of Florida, Doug is
retiring to relax and pursue new hobbies and adventures.
Beloved by university
students, geoscientists and all his other co-workers and customers, Doug is known for his strong desire for service and a willingness to assist
library guests and researchers with scientific articles of interest. With vast
experience as a science-technology librarian and computer network
administrator, Doug’s work has been invaluable to all geological research
projects at the FGS, while his services were also available to other DEP
geoscientists as well as to the public.
After obtaining a B.A. in
Geological Sciences and a M.L.S. in Information Science, Doug was hired in
September 1977 as a librarian for the state of Florida’s Department of Natural
Resources/Bureau of Geology (now the Florida Geological Survey). After three
years with the Bureau of Geology, he worked for 17 years at Florida State
University as an associate university librarian at the Dirac Science Library. During
this time, he found teaching new students about the importance of using
scholarly sources (and citing them properly) very rewarding. In 1997, Doug
gained computer networking experience as a computer systems administrator for
UNC-Greensboro SERVE Education Lab before moving to Washington state to work as
a science librarian at Washington State University’s Owen Science &
Engineering Library. During this time, Doug taught graduate and
undergraduate level courses, which included subjects related to computer
science, mechanical engineering and materials science.
In 2006, Doug
returned to Tallahassee and filled the vacant librarian specialist position at
the FGS Herman Gunter Library. Doug’s experience and interest in working with
scientists in an atmosphere of scientific research, discovery and publication
made rehiring him the right decision.
|
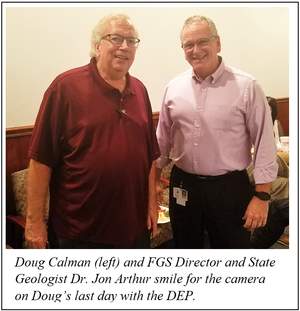
As an integral part of
the initial phase in most research proposals or projects, Doug conducts a
literature review of published research. He routinely provides updates on
library and reference resources to employees based on their research areas and
interests and sends all FGS employees frequent emails on library
resources. He has contributed significantly
to DEP/FGS research productivity by communicating relevant information in
assisting a research project.
In addition, Doug
maintains several important reference databases in the various responsibility
areas of FGS research scientists, and continuously adds to the FGS publications
and geological information available to the public online, improving on
quality, resolution and ease of use. These crucial services provided to the
department earned Doug recognition over the years, including FGS Employee of
the Year in 2010 and DEP Employee of the Month in November 2011.
During Doug’s career at
the FGS, he cooperated with the Leon Advocacy and Resource Center to provide
work experience to students in the Leon County Schools’ Exceptional Student
Education program. The students involved in this cooperative project scanned
valuable paper-copy geologic descriptions of well samples. Doug’s willingness
to work with challenged students and their teachers gave the students an
opportunity to contribute and learn while also making valuable DEP/FGS data
available to the public. He also participated in FGS outreach events and was
always available to package and provide relevant FGS publications to the public.
Doug has been a delight to
work with, and the FGS wishes him success, happiness and good health in his
retirement.
Contact: Sarah Erb, OMC Manager
|
|